Test story - Format
Invention is not just genius or inspiration. It’s a process that can be analyzed, using historical cases to understand how inventors work.
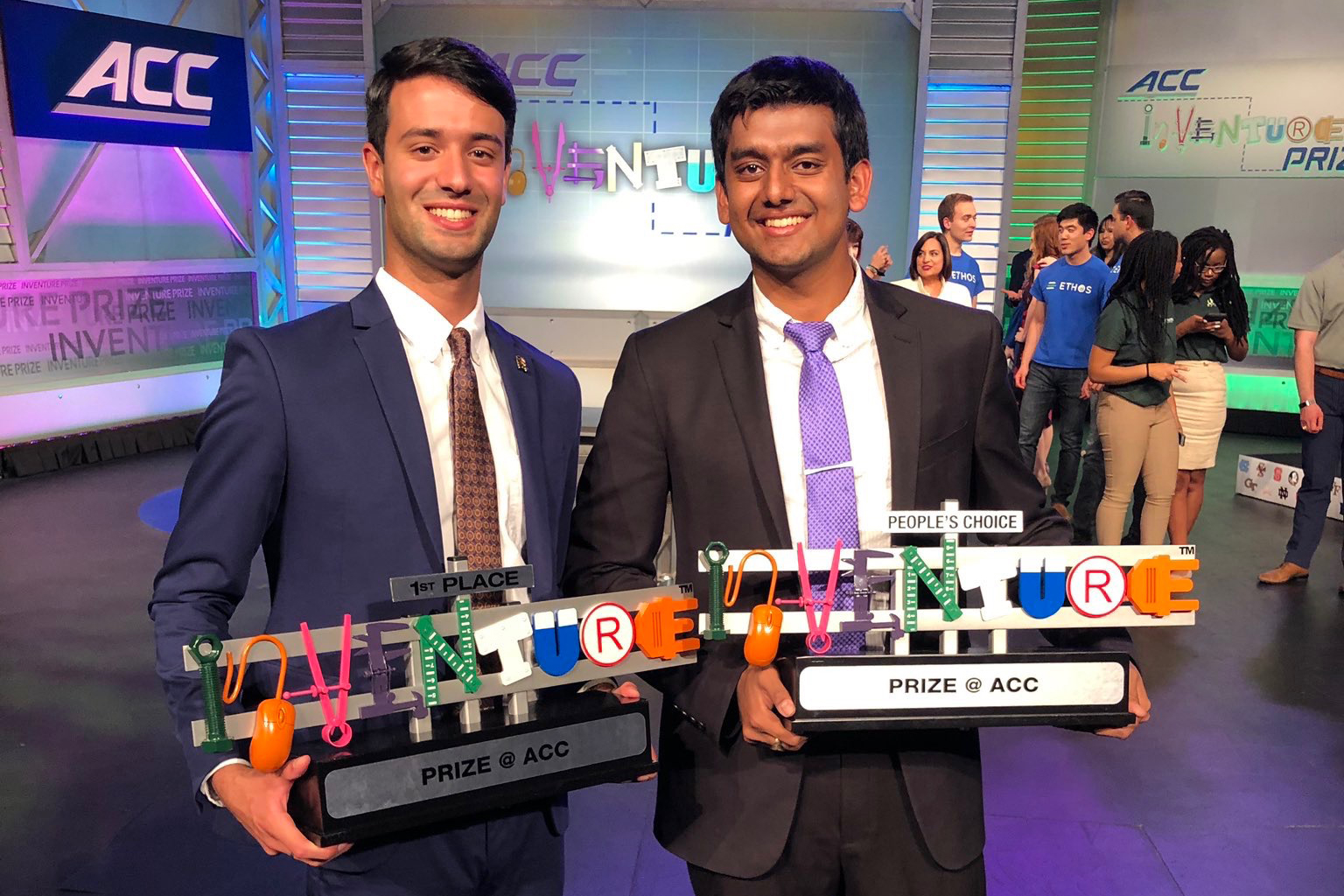
Editor’s Note: Professor Bernard Carlson is Chair of the Department of Engineering and Society in the UVA Engineering School, author of several books, and editor of the definitive, seven-volume Technology in World History.
Many people do not appreciate what history can teach us about invention and innovation. Great inventions, it is commonly assumed, come only to incredibly smart people like James Watt, Nikola Tesla, or Steve Jobs — these individuals possessed exceptional mental abilities that allowed them to do the extraordinary. Many people tend to think of invention as a mysterious, unknowable activity. As much as we would like to characterize the brain as a computer, we cannot fathom how humans are able to create beautiful paintings, amazing scientific theories, or inventions that revolutionize daily life.
If you regard invention and innovation as the products of genius, luck, or mystery, it is easy to conclude that innovation cannot be taught: you are either born to be the next Thomas Edison, or you are not. Invention — like all creative acts — is not something that you can teach; you can nurture and inspire the next Elon Musk, but you cannot reduce invention to ten easy lessons. To teach invention, then, is to teach the unteachable.
To teach students how to be innovators, we study the process of great inventors.
Despite these popular notions, my colleagues and I at the School of Engineering and Applied Science at the University of Virginia have been working to teach the unteachable to students for thirty years. We have approached invention as a process that can be analyzed, using historical cases to create a robust notion of how inventors work. Moreover, we have distilled principles and techniques to teach students how to be innovators.
Much of my professional life has been devoted to studying such major inventors as Thomas Edison, Alexander Graham Bell, and Nikola Tesla. Inspired by historian Thomas P. Hughes, I decided to study inventors because they often left substantial source material (notebooks, letters, testimony, and artifacts). And because nonacademic audiences are interested in inventors, you can use inventors to talk about significant social and ethical issues relating to technology.
I began studying inventors in the early 1980s by examining Elihu Thomson, a contemporary of Edison. Along with inventing a successful arc-lighting system and doing pioneering work with alternating current, Thomson was significant because, unlike other late nineteenth-century inventors who generally worked alone, he spent his career in a large company, General Electric. This gave me the opportunity to look at how the organizational environment affects the innovation process.
Thomson’s career showed that innovation is a social process not only in the sense that it involves the interplay of individuals and groups, but also because effective innovation requires the coproduction of machines, financial arrangements, and markets.
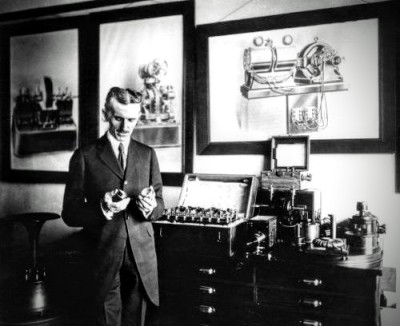
But as I read through Thomson’s letterbooks, filled with memos he wrote to vice presidents, I realized that while I was learning a lot about how he moved his inventions through the company, I wasn’t learning as much about how he conceived of his inventions. Thomson viewed dictating letters as being one step removed from the creative work on the benchtop, and I came to agree with him. How could I get closer to the point of knowledge and artifact production?
To investigate more closely what inventors did at the benchtop, I began collaborating my colleague Michael Gorman. Gorman is a cognitive psychologist who had been conducting simulations of how people solve scientific problems, and he welcomed the idea of using historical materials to investigate how people developed new technology. Together we chose to look at the invention of the telephone because of the availability of substantial archival materials on Alexander Graham Bell, Elisha Gray, and Thomas Edison.
We undertook a fine-grained examination of work at the inventor’s benchtop, and to do so, we turned to a Gorman’s area of expertise, cognitive science. A dominant issue in cognitive science in the late 1980s was the tension between mental models and heuristics. Some major figures in the field — such as Philip Johnson-Laird — believed the key to understanding how people think was to comprehend the meta-ideas, or categories, by which they processed information, what cognitive scientists call mental models. Other researchers—such as John Anderson and Herbert Simon — argued that cognition is more about the strategies or procedures employed in thinking. These strategies and procedures are called heuristics.
We decided to explore how inventors might use both mental models and heuristics in their work. At the same time, we wanted to pay attention to the specific objects an inventor manipulates on the benchtop. After all, it seemed possible that inventors might implement their mental models with specific mechanisms, circuits, or materials with which they were familiar. Edison, for example, took the drum cylinder he had used on the phonograph, covered it with a photographic emulsion, and invented the kinetoscope, his motion-picture device. To pay attention to these “building blocks,” we added mechanical representations to our analysis.
By comparing how Bell, Gray, and Edison each worked on the telephone, we produced generalizations about how they invented.
With these categories in mind — mental models, heuristics, and mechanical representations — we set out to study the notebooks, sketches, models, patents, testimony, and correspondence of our three inventors. We hoped that by comparing how Bell, Gray, and Edison each worked on the telephone, we could produce generalizations about how inventors worked, generalizations that could be tested further by studying other inventors.
As we started to study the primary sources, Gorman and I quickly realized that verbal accounts (such as patent testimony) did not always square with the visual and physical record (notebooks, sketches, and artifacts) produced in working on an invention. Verbal accounts of the invention process were typically created years later when an inventor was seeking to prove that he or she was the first to invent something. Adhering to the popular notion of a eureka moment, inventors often collapse the long process of trial and error into a single clairvoyant moment of insight.
If we were going to get close to what our inventors were doing at the benchtop, we needed to create techniques for analyzing the visual as well as the written materials. Given the volume of sketches (dozens for Bell and Gray, hundreds for Edison), we decided to develop a computer-based system for organizing and storing the visual sources.
At this point, Gorman and I took advantage of the fact that we teach undergraduate engineering students at UVa. We turned to our best students for help. Over a seven-year period (1989-1996), they helped us devise a mapping technique for analyzing the visual materials used by our inventors.
One way to understand our mapping technique is to think about the workspaces of inventors and artists. If we look around an artist’s studio or an inventor’s laboratory, what do we find? Both creative spaces are crammed with models, sketches, and notes as well as machines or paintings in various stages of development. Using all these resources, an artist or inventor is trying to merge ideas in the mind (mental models) with objects in the real world (mechanical representations).
While it is easy to picture an inventor tinkering with a device on the benchtop, this is not the only way that he or she can mingle ideas and objects. He or she can do the same with a variety of substitutes; rather than make a full-scale version of a device, an inventor might use a prototype, sketch, calculations, computer simulations, or even a written description. The advantage of these substitutes is that they often can be generated more quickly and manipulated differently than the full-size device. Edison, for example, filled notebooks with sketches because drawing allowed him to determine quickly whether a device would work, thereby allowing him to avoid the cost and hassle of building each version that he envisioned. Substitutes that stand in place of the fully developed invention — such as prototypes, sketches, calculations, and written descriptions — are representations of an invention.
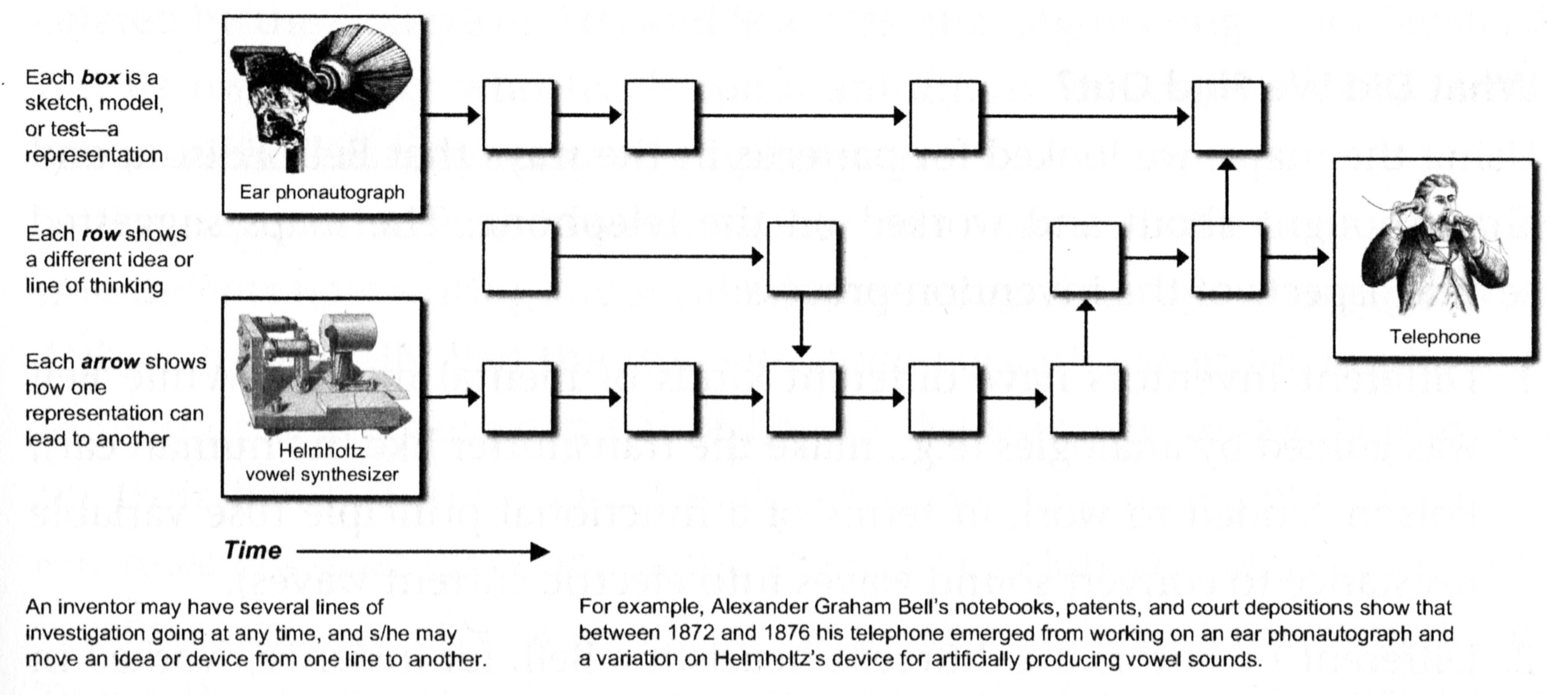
Invention, then, can be considered an activity in which inventors use a variety of representations to merge an abstract idea in their mind with material objects that exist out in the world. One way to sort through these representations is to create a map. Suppose you allow each permutation of an invention — each prototype, model, sketch, or experiment — to be a box on a map. This “box” can be a piece of paper, a square drawn on a computer, or a Post-it on the wall; it doesn’t matter. In our case, we scanned all three inventors’ sketches into the computer and then cut-and-pasted them into the boxes on the map. We frequently gained insights by placing several sketches side by side on a map to look for similarities and differences.
Once you have the boxes, you can then place them in chronological sequence on a map in which time moves horizontally from left to right. Arraying the representations in chronological order allows you to look for cause and effect. In some cases, one sketch prompts an inventor to produce another sketch or model, and you can capture these connections by drawing arrows between the boxes.
You can also arrange the boxes in different rows, with each row representing a line of investigation or particular invention. Inventors frequently pursue several lines of investigation simultaneously and may move an idea or device from one line to another. For instance, when Edison was working on the telephone in 1877 and 1878, he was simultaneously investigating his quadruplex telegraph as well as the phonograph. In fact, a key aspect of creativity is the willingness to move ideas and objects from one line to another — to mix things up in unexpected ways.
What Did We Find Out?
Using the maps, we looked for patterns in the ways that Bell, Edison, and Gray worked on their telephone inventions. The maps revealed several aspects of the invention process:
- There are different kinds of mental models. While Bell was guided by analogies (e.g., make the transmitter like the human ear), Edison tended to work in terms of a functional principle (use variable resistance to convert sound waves into electric current waves).
- Different inventors use different heuristics. Bell, for instance, worked in a very methodical fashion, testing each possible variation of his basic design on the benchtop. In contrast, Edison often varied several parameters at once and did not conduct as many benchtop experiments, because sketching allowed him to determine if a particular design would work.
- While Edison generally worked “bottom up,” manipulating mechanical representations to formulate his mental model, Bell worked “top down,” starting with a mental model that he then tested on the benchtop.
Teaching Innovation
As we discerned patterns in our maps, both Gorman and I began to apply what we were learning to teach our engineering students how to invent. Gorman, for instance, teamed up with a colleague in mechanical engineering, Larry G. Richards, to offer a course on invention and design. In early versions of this course, students constructed their own telegraph and telephone systems. Their course had a profound impact on one student, Evan Edwards, who with his brother Eric, invented an auto-injector for medicines, now known as AuviQ.
While Edison generally worked “bottom up,” manipulating mechanical representations to formulate his mental model, Bell worked “top down,” starting with a mental model that he then tested on the benchtop.
Meanwhile, I transferred ideas from our research to teaching communications to first-year engineering students. Although I initially had the students study Edison’s career for inspiration, I soon switched to having the students imitate Edison and try their hand at invention. I borrowed two fundamental ideas from our research — that invention is the interplay of ideas and objects and that inventors mix up ideas and objects by using a variety of representations.
To get objects into the classroom, I had students build kits, first a pendulum clock and then a robot car. For the representations, I had them sketch ideas in notebooks, write technical descriptions, and ultimately draft a patent application. Through this teaching, I came to agree with the great cognitive scientist Herbert Simon, who insisted, “Solving a problem simply means representing it so as to make the solution transparent.”
Adding Context to the Cognitive Framework
Yet I continued to mull over what Gorman and I had learned about the invention process. In particular, I was troubled that our cognitive framework did not take into account the social, economic, or cultural context in which inventors work. How might larger, external forces be included in this cognitive framework?
To teach invention and design, engineering textbooks often present these activities as a linear model wherein ideas move in a straightforward, non-problematic path from conception to manufacturing and adoption. (figure X). This model would suggest that inventors move through a specific sequence of steps with no false starts or backtracking. To me, the linear model embodied several dichotomies:
- It assumed that ideas come before devices, yet some inventors work closely with devices first and in so doing generate new ideas.
- Social factors appear only at the beginning and end of the model, yet inventors may be influenced by external factors throughout their work.
- The model drew a distinction between the technical occurring in the middle and marketing, which comes at the end. But inventors are often doing both technical and marketing work at the same time.
While a linear model may be useful for introducing students to design, it is problematic for understanding how inventors actually work. For scholars studying invention, this sort of one-size-fits-all prescriptive model can seem like jamming a square peg into a round hole.
While a linear model may be useful for introducing students to design, it is problematic for understanding how inventors actually work.
I wondered if it would be possible to build on my work with Gorman to create a model that would soften these dichotomies; could we create a nonlinear model that would capture the ways that invention is both cognitive and social?
Rethinking Invention as a Process
I began by reconsidering what it means to say that invention is a process (figure 16.3). Like other processes, invention has both inputs and outputs. In terms of inputs, what does an inventor use in the course of his or her work? Besides tangible resources (money, tools, and materials), he or she also relies on other individuals to provide help with such essential tasks as model building, patenting, manufacturing, and marketing. And an inventor draws on a range of intangible resources, including preexisting knowledge (from both science and other fields), legal expertise (patents, regulations, and contracts), and emotional support. Inventors may also be influenced by public events (such as wars or business conditions) or their personal situation (love, marriage, health, emotional depression), and so these should be included as inputs.
In terms of outputs, we expect inventors to produce devices and patent applications, but they also need to promote their creations. Hence, the outputs from the invention process should include interviews, lectures, and publications. And since inventors may sell or license their creations, we should also include business deals.
While inventors can bring some resources into the creative process on their own (for instance, they can read the technical literature or study needs in the marketplace), these inputs and outputs often come into the invention process through interaction with other people. These individuals can include business partners, skilled assistants, family and friends, patent lawyers, and newspaper reporters. All of these intermediaries affect how ideas, resources, and results flow in and out of the invention process.
By considering the inputs and outputs to the invention process and by highlighting the individuals with whom inventors interact, I was placing the cognitive work of invention into a social context. Including an inventor’s relationships in the diagram allowed me to show how inventors draw on their social environment and, at the same time, how they seek to shape it.
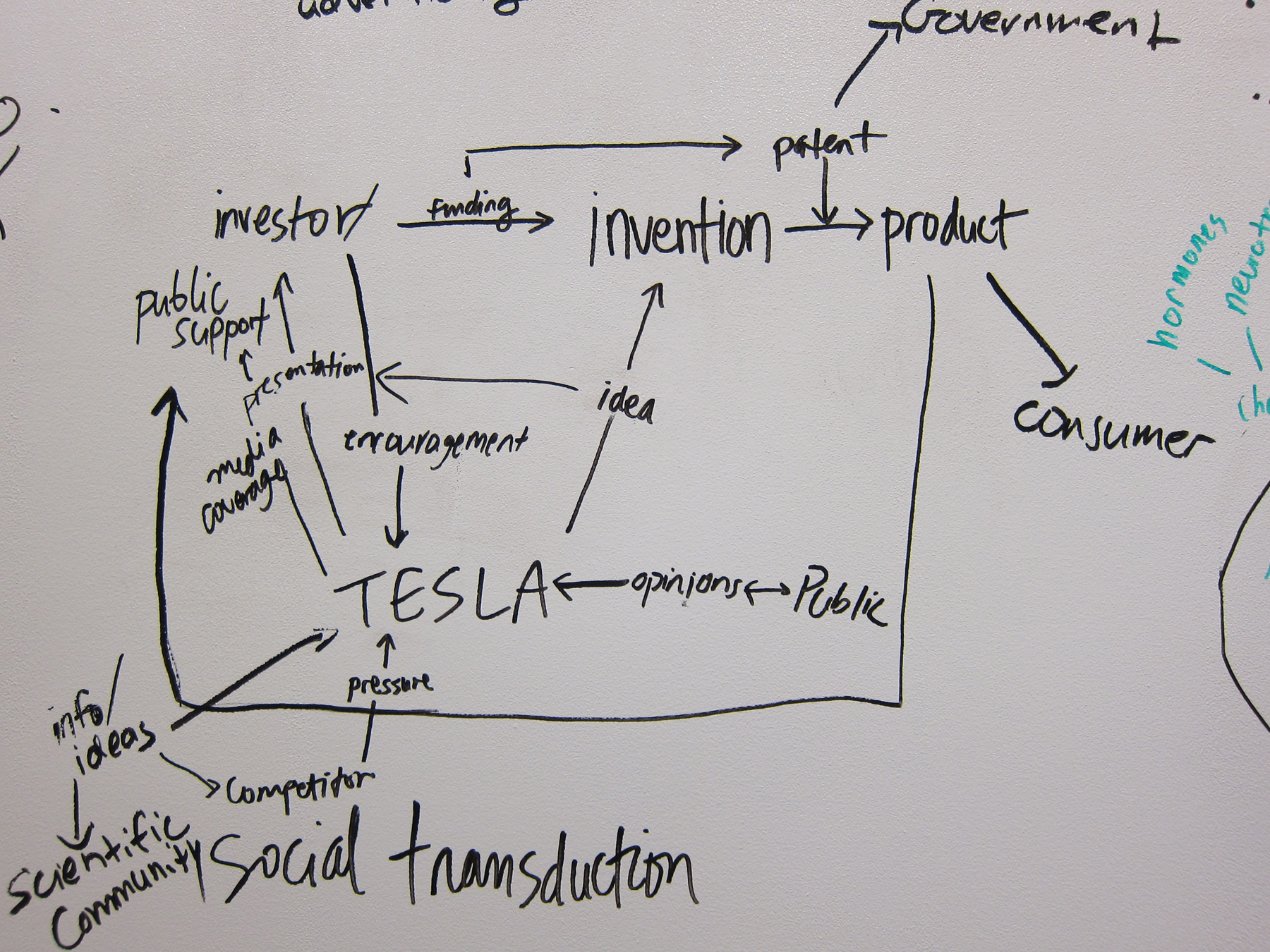
An Inventor’s Flow
As I articulated invention as inputs-process-outputs, I soon realized that what Gorman and I had worked out in our research on the invention of the telephone was all the stuff that belonged in the middle, in the process box on figure 16.3. We had been mapping the activities by which an inventor gets an idea out of his head, refines it through multiple representations, and realizes it on the laboratory bench.
What I had not yet appreciated was that invention is not just a process but a state of mind. Like other creative people, inventors intentionally strive to generate a steady stream of ideas and representations and to shape these ideas into meaningful inventions. Psychologist Mihaly Csikszentmihalyi calls this effort to generate a steady stream of ideas the “flow” of creativity.’ Taken as a whole, mapping the various representations used by inventors gives us a visual picture of the flow of their work.
Like other creative people, inventors intentionally strive to generate a steady stream of ideas and representations and to shape these ideas into meaningful inventions
In looking at an inventor’s flow, it is important to consider short-term versus long-term goals. Immersed in the creative process, an inventor may not be worried about the ultimate version of an invention but rather be thinking about how to move from one representation to the next. Being in the flow means being focused on the immediate opportunities and open to new possibilities as they present themselves. Along the way, inventors may change their mind about the ultimate goal, or the goal may only become clear by doing, by being in the flow. Hence, while it would be tempting to create a map of the invention process in which everything funnels into what we know as the final version, we should instead strive to include the ideas and devices that went nowhere, the false starts, and the wrong turns.
Combining Process and Flow
I now brought these two perspectives—input/output and flow—together on a single page (figure 16.4). I let the process move vertically down the page, with the inputs at the top and the outputs at the bottom. Meanwhile, I had the inventor’s flow move horizontally across the page. This diagram allows us to follow how ideas and resources affect an inventor’s flow as well as how prototypes and other results then move out from the inventor’s workshop into society.
In organizing the inputs and outputs depicted in figure 16.4, it is important to arrange them by how they are experienced by the inventor, rather than by some sequence embedded in the model. This is the major advantage of the mapping technique over the old linear model. For example, the inputs—ideas, encouragement, needs, resources, and events—should be placed in the order by which historical sources indicate they affected the invention flow. In situations where a version of an invention is reported in a newspaper or written up in a patent application, we can connect a box in the flow that indicates outputs. Rather than squeezing the historical materials into the model, the model and diagrams are modified to reflect what the inventor was doing.
Yet we should be clear that the story portrayed in the historian’s flow process model can be very different than the one an inventor might tell about creating a particular device. It is important to recognize that inventors may edit the flow process down to a simple narrative to secure strong patents and claim that there was a logical progression in their thought processes. Moreover, an inventor may simply not be able to reconstruct all the twists and turns he took on the way to a particular outcome. Edison, for instance, introduced hundreds of sketches of the telephone as evidence in patent litigation in the late 1870s, but in testifying, he couldn’t begin to explain the evolution of his thinking.
What Did This New Model Capture?
The flow model helps us capture three aspects of the invention process:
- The people, ideas, and resources that shape an inventor’s work
- The activities that inventors use to develop new inventions (thinking, sketching, experimenting, and testing)
- The results of the invention process (patents, prototypes, processes, products, and publicity)
It is important to note that this model is not intended to be restrictive. No “one size” fits all inventors. Indeed, the point of the model is to draw out the unique and special qualities of each inventor. The model serves to help us ask consistent questions across different episodes of invention. It should help us discern both the social and the cognitive dimensions of creativity. But, above all, this new model should enlarge our vision of What invention is and challenge us to better understand how people can create remarkable technology that changes the world.
It is important to note that this model is not intended to be restrictive. No “one size” fits all inventors.
The Entrepreneurial Turn in Research and Teaching
Thinking about invention as a nonlinear flow process proved to be enormously helpful in writing a biography of how Nikola Tesla worked, but this new model once again altered my teaching. One of the most powerful features of the model is that it reveals the intellectual and social work required to convert a benchtop device into a commercial product. Left on their own, inventors often prefer to concentrate on the flow, on moving from sketch, to model, to new experiment; in other words, inventors want to keep moving in a horizontal direction.
When the time comes to convert a benchtop device into a commercial product, however, the inventor must change direction; rather than put his or her energy into generating new variations, he or she has to work on connecting an invention with the wider world via publicity, business deals, or manufacturing. Often these connections cannot be made by the inventor alone but require the assistance of partners and patent lawyers. On the diagram, the movement of an invention from the benchtop to the marketplace means shifting from the horizontal to the vertical. Hence, the diagram shows major decisions — where the technical gets connected to the social — as a turn.
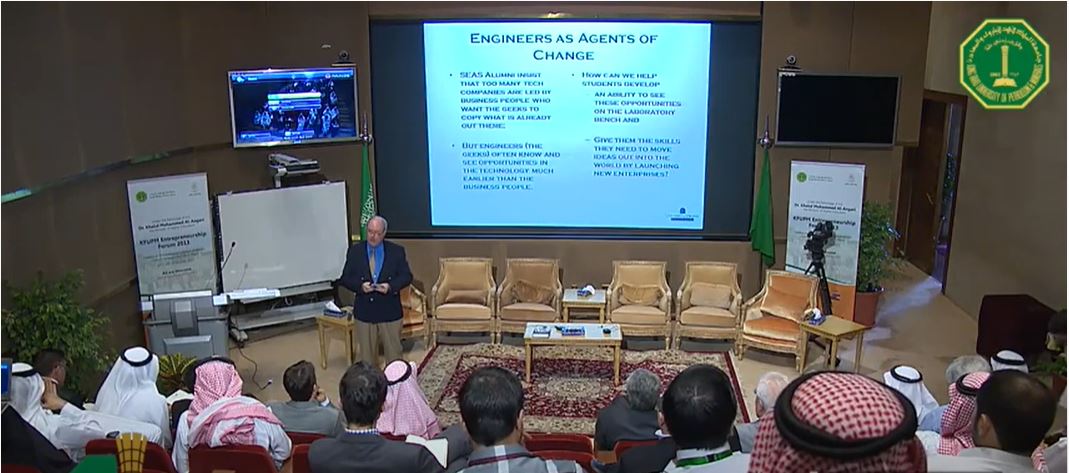
This entrepreneurial turn brings into crisp focus the importance of partnerships in technological creativity. Careful observers will notice that partnerships combining technical skills with business acumen are scattered across the history of technology. In the case of Tesla and his AC motor, he needed the help of his business partners, Charles Peck and Alfred Brown, to know which motor ideas to patent, demonstrate in public lectures, and ultimately sell to George Westinghouse.
The notion of an entrepreneurial turn also had powerful implications for teaching innovation. Several key ingredients are required to bring innovations into the world:
- There must be creative people — inventors, designers, engineers, and scientists — who are allowed to generate ideas and prototypes. The British lovingly call these creative types “boffins.”
- The boffins must be protected from external distractions so they can be in the flow. This may mean giving them their own resources and space in an organization.
- At the same time, there must be individuals — entrepreneurs — who can move the most promising variations from the flow and move them out into the world.
Many people believe the most promising future start-ups would not be led by MBAs who employ engineers, but rather by engineers who know about entrepreneurship.
These three ingredients became, first, the kernel of a new course, and then a new program at UVa.
In a new class, “Engineers as Entrepreneurs,” I guided students as they explored the habits and practices required to place themselves in a creative flow: how to observe the world; represent it in words, sketches, and simulations; and generate innovations. My students had to understand that at times engineers — like artists or musicians — must fully immerse themselves in a technically rich stream of activities. At the same time, I challenged them to pay attention to what customers and other stakeholders might need or want by conducting interviews and market research.
“Engineers as Entrepreneurs” was well received by UVa students and caught the attention of engineering alumni who wanted to promote innovation within the school. Working as venture capitalists in Silicon Valley and elsewhere, several alumni had learned that the most important people to meet in a startup were not the “suits” (the MBAs), but rather the boffins, the engineers. Indeed, these alumni had become convinced that the most promising future start-ups would not be led by MBAs who employed engineers, but rather by engineers who knew about entrepreneurship.
With guidance and financial support from these alumni, we launched UVa’s Technology Entrepreneurship Program (TEP) in 2010. The program offers several undergraduate courses that students take as part of their engineering major or in pursuit of a minor in entrepreneurship. In these classes, students work in teams and practice pitching their ideas to investors and entrepreneurs from the region. TEP also provides cocurricular activities and a dedicated workspace to help students meet outside of class to brainstorm, form teams, and network with local entrepreneurs. Alumni have continued to support the program by endowing several funds that support student projects.
The big idea guiding TEP is that entrepreneurs need a community around them. Popular mythology often depicts entrepreneurs, like inventors, as solitary individuals working alone. However, as the flow model reminds us, inventors rely on friends, family, assistants, and investors to be creative. In the same way, we have found that entrepreneurs need to interact with other a variety of people who provide ideas, guidance, and motivation. Across history, at the heart of the most innovative economies, you will find communities of entrepreneurs — whether it be Amsterdam’s coffeehouses in the seventeenth century, Philadelphia textile mills in the nineteenth century, Detroit machine shops in the early twentieth century, or Silicon Valley in the late twentieth century. In each of these places, entrepreneurs gathered to share and support one another.
By drawing on history and listening to our students, we are nurturing a community of entrepreneurs. We not only introduce students to basic ideas about entrepreneurship, but we go out of our way to mentor those students who are ready to launch an enterprise. We are especially proud that our founders frequently place highly in entrepreneurship competitions; for the past several years, our student teams have finished first in UVa events, including the E-Cup and the Darden Business Plan Competition. In 2016, Contraline, a student team whose members had invented a new contraceptive for men, placed second in the Atlantic Coast Conference’s InVenture Prize. Another student team, Agrospheres, won the 2016 Collegiate Inventors Competition and took top place in the 2017 InVenture Competition. Agrospheres has since raised $750,000 to support development for using nanospheres to deliver pesticides precisely and safely to crops. And in 2018, Ashwinraj Karthikeyan not only won the ACC Inventure Competition again for UVa but also received the $50,000 Pike Prize for Engineering Entrepreneurship. Karthikeyan has developed a new bandage for treating diabetic foot ulcers and he will be deploying it in India.
By drawing on history and listening to our students, we are nurturing a community of entrepreneurs.
Conclusion
How should innovators be trained? Can you use the past to teach the supposedly unteachable? What we have learned is that invention and entrepreneurship are not simply about genius, luck, or confidence. By studying Edison, Tesla, and Bell, you can find patterns in the ways they thought and worked. Using these patterns, you can formulate a framework that helps students to invent and launch ventures.
If you want students to be innovative, they need to know that invention is the interplay of ideas and objects. They need to learn and practice using different representations — notes, sketches, prototypes, and simulations — to move ideas from the mind’s eye into the material world. We can help them understand that inventors seek to be in a creative flow, striving to learn from each representation and to keep generating new variations. And student innovators can master the entrepreneurial turn — what it takes to recognize the most promising idea in a creative flow and to guide it from the laboratory bench to the end user. The past can indeed be used to create curricula for innovation and entrepreneurship.
Ultimately, what we teach is a mindset, an attitude that change is possible. Our students know that they can develop the skills needed to innovate. Innovation, at its heart, is about nurturing the human spirit and fostering a faith in progress. None of our students have gone on to become the next Nikola Tesla — yet — but we see signs that we are on the right track.